In this iBiology lecture, Prof. Xiaowei Zhuang (Harvard, HHMI) provides an overview of Super-Resolution Fluorescence Microscopy, with a special focus on STochastic Optical Reconstruction Microscopy (known by the acronym STORM) which was developed in her lab. STORM overcomes the diffraction limit by using photo-switchable fluorescent probes to temporally separate the spatially overlapping images of individual molecule. Different subsets of fluorescent probes are activated at different times, allowing these fluorophores to be imaged without substantial spatial overlap and to be localized with high precision. Iterating the activation and imaging process allows the position of many fluorescent probes to be determined and a super resolution image can be reconstructed from the positions of the localized probes.
In this iBiology lecture Dr. Jennifer Lippincott-Schwartz (NIH) begins by showing the resolution limits of conventional fluorescence microscopy (about 400nm to tens of microns). Molecular structures are often below this 400nm in size. Super resolution systems allow us to acquire images in nanometric region from a few nm -to- ~400nm. She further illustrates the problem with conventional fluorescence microscopy by describing how a GFP molecule of 2.5nm (diameter) size would appear when imaged with a microscope with a PSF that is ~100x the size of a GFP molecule. With several molecules in close proximity, it would be impossible to discern how individual molecules are distributed within the blur of the PSF.
Dr. Lippincott-Schwartz then shows how we can apply curve fitting methods, using our knowledge of the Gaussian nature of the PSF. In this way we can determine, to a fairly high degree of accuracy, where a particular molecule might be located.
The localization problem becomes more complex when more than one molecule is involved. In the next part of the lecture Dr. Lippincott-Schwartz describes how the ability to switch on and off molecules presents a method by which one can track individual molecules in a fixed sample with many molecules. She focuses on Photo Activated Localization Microscopy (PALM), a process that allows the behavior of individual fluorescent molecules to be followed.
It is worth noting that Dr. Lippincott-Schwartz and her colleague Dr. George Patterson were the inventors of the original Photo- Activated GFP and that it was in her lab at NIH that the first super-resolution PALM microscope was invented by Nobel Laureate Eric Betzig and Harald Hess.
In this iBiology lecture Dr. David Agard (UCSF, HHMI) describes several methods for going beyond the diffraction limit of an optical microscope.
He begins by outlining the problem in terms of the finite NA of the objective. He goes on to describe methods by which to “cheat” physics and obtain a higher resolution that available with conventional methods.
In the first method, we collect more of the information that could be collected with one objective. By using two objectives, we get an effectively higher NA, nearly doubling the achievable resolution. With the sample wedged in between two objectives, we can use the light from both objectives by ensuring that they interfere with each other. He also shows how we can get an even higher effective NA by modifying the system to achieve simultaneous illumination and emission, creating interference patterns for both the light going in and for the light coming out of the sample. An additional benefit, although at the cost of some complexity, is that we get a much higher axial resolution than previously possible.
In Structured Illumination Microscopy (SIM) instead of illuminating the sample with a uniform field, we use a patterned illumination field. The interaction of the patterned illumination field and the structure of the sample produces fringe patterns that effectively transform the higher spatial resolution content of the sample into information at a lower spatial resolution, within the imaging capability of the objective.
This technique requires computation to extract the higher spatial frequency information from the resulting image.
In this iBiology lecture Nobel Laureate, Dr. Stefan Hell, (Max Planck Institute for Biophysical Chemistry) outlines the need for Super Resolution Microscopy and focuses in detail on two special techniques.
He begins by showing the importance of light microscopy that can approach the type of resolution that was previously only available via electron microscopy. He describes the “focusing problem” with light microscopy, as first discovered by Ernst Abbe. Essentially one is unable to create a point of illumination due to diffraction – instead, there is a ~200nm (dia) zone of light that is flooded by light. Within this zone, we cannot discern individual features because all the molecules in the zone are emitting light.
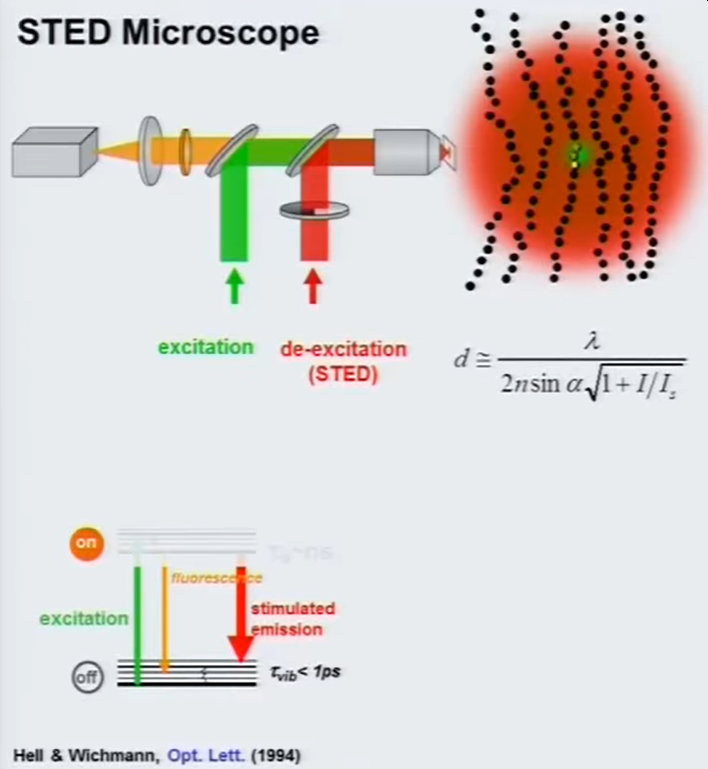
In STED [Stimulated Emission Depletion] microscope we use two beams. One of the beams is used for excitation and the other donut shaped beam serves is to keep certain molecules silenced, essentially preventing them from emitting light. This “red-shifted” beam adds photons that don’t have an energy that is high enough to excite molecules. These photons are capable of sending the If the energy level fits the energy gap between the fluorescent state and the ground state of the excited molecules, they no longer emit light.
The second half of this video is on the topic of RESOLFT [REversible Saturable OpticaL Fluorescence Transitions]. This is a technique that uses the same ON/OFF technique as in STED, but at much lower light levels which is essentially more gentle on the samples being imaged. We utilize the two reversible metastable [cis-trans isomerization] states of fluorophores with relatively long lifetimes, from several milliseconds to seconds. Since these lifetimes are relatively long, they require much less optical energy than in a STED setup. This technique has become more popular with the advent of new photoswitchable fluorescent proteins which can accommodate many ON/OFF cycles.
Cameras recommended for Super Resolution Fluorescence Microscopy: sCMOS Cameras